NSF: EPSCoR Kampers
Kentucky EPSCoR project KAMPERS (Kentucky Advanced Manufacturing Partnership for Enhanced Robotics and Structures) aims to develop global excellence in next-generation flexible electronics, robotics, and manufacturing technologies. KAMPERS will advance the state-of-the-art in advanced manufacturing through integration of synthetic biology, 3D printing, and the emerging field of printed electronics to revolutionize the capabilities of robotic manufacturing infrastructure, medical assistive robots, prosthetics, and consumer products.
The KAMPERS team includes faculty, staff and students from eight universities and colleges across the state of Kentucky.
Our research team at the University of Louisville includes a group of 11 faculty from Electrical, Mechanical, Computer Science and Bio Engineering at Speed School, and two faculty from the Biology Department ofthe School Arts & Sciences. They are collaborating with colleagues at UK and several other regional universities including EKU, WKU and MoSu. Our team includes 11 Ph.D. students, 5 undergraduate researchers and 5(?) postdoctoral associates. In addition to the defined research thrust objectives enumerated below, we are also working to deploy robots to combat the spread of Covid-19 around medical facilities in the very near future and have been experimenting with incorporating UV and sprayers for disinfection.
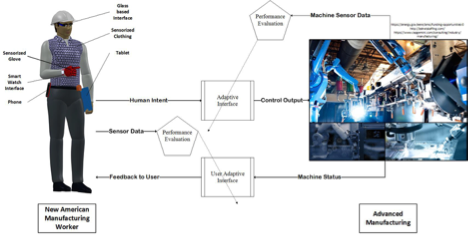
Research Thrust RT-1
Develop new sensing modalities designed to be integrated into structural robotic components, along with the multi-functional materials required to serve as electronic interconnects and insulators. This thrust develops the materials and fabrication processes needed to embed electronic function into structural components, and involves developing new materials, device configurations, and structural forms for demonstration of basic logic, sensing, and data processing arising from co-printed electronic and structural elements, along with on-board power generation and storage. Explore synthetic biology approaches to yield structural materials with programmable lifetimes, to reduce generation of persistent electronic waste.
Objective 1.1.2: Explore to what extent new materials synthesized through synthetic biology can yield superior structural polymers or resins with programmable lifetimes. (Running, Schultz)
Model Systems for production of bio-renewable materials
Tobacco
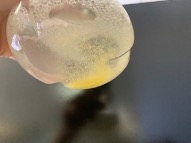
Extensively studied model organism
Easy to culture
Carrot
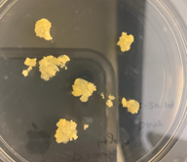
Monoene Metabolic Platform
Geranium
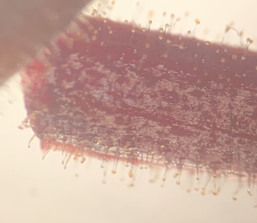
Anacardic acid Metabolic Platform
Moss
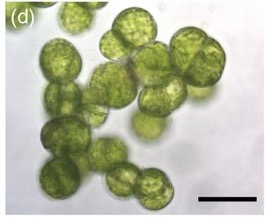
Extensively studied model organism
Currently used in bioreactors
Objective 1.3.3: Develop structurally integrated biological and environmental sensor demonstrators, with potential programmable structural lifetime. (Roussel, Welch)
Objective 2.1.2: Develop and formulate fused filament, composite fiber, in-situ polymerizable monomers, and/or thermoplastic conductors and semiconductors amenable to printing. (Kate, Harnett)

Objective 2.1.4: Develop, optimize, and demonstrate multi-material printing processes for structural electronics with robotic and wearable manufacturing applications. ( Kate, Harnett, Popa)

Objective 2.2.2: Integrate communication and data storage components and develop operating system software for electronically augmented structures with sensing and logic functions. (Naber)
Industry Collaborator: Biolink LLC
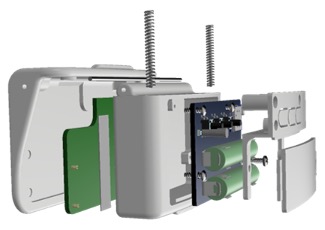
Research Thrust RT-3
Develop enhanced, adaptive software and feedback modes to maximize impact of increased sensing capabilities and bring collaborative Human Machine Interfaces (HMIs) to the level needed for advanced manufacturing and health care applications. Utilize extensive sensor data from structures made in Thrusts 1 and 2, create the next generation of Collaborative Human-Machine Interfaces (CHMIs), broadly defined as the intelligent connection between novel multimodal arrays of sensors monitoring users and the environment, and collaborative control decisions and actions taken by machines to assist their human users.
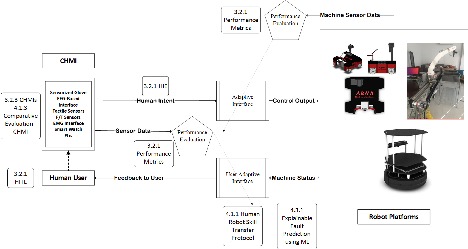
Objective 3.1.1: Develop design, manufacturing and scalability protocols for fiber-based electronic textiles. (Harnett, Kate)

Figure 1 gives an overview on the current work and future goals to use conductive short/long continuous fibers, also a proposed plan to have functionalized coating for property enhancements has been illustrated which will be undertaken during the upcoming year.
Objective 3.1.2: Configure functional sensor surfaces for human activity detection in manufacturing and healthcare applications. (Roussel)
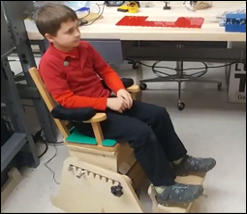
Objective 3.2.1: Select performance metrics for human-in-the-loop utilizing large numbers of multi-modal sensors for robots or collaborative human-machine interfaces. (Welch, Popa, , Nasraoui):
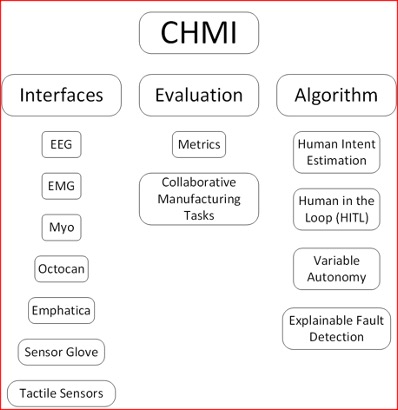
Objective 3.2.2: Develop new control techniques for human-in-the-loop (HITL) collaborative robotic systems. (Popa, Welch, Nasraoui)
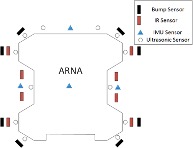
Figure 3: Adaptive Robot Nursing Assistant (ARNA)
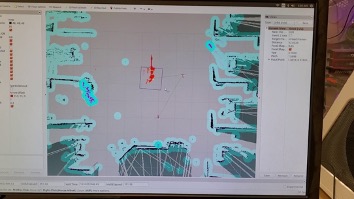
Figure 4: Interface for Autonomous Navigation
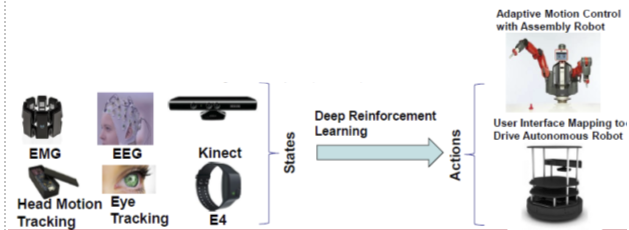
Objective 3.2.3: Develop brain-machine interface (BMI) protocols for interactive modulation of sensorimotor rhythms. (Sunderam, Popa, Harnett, Roussel)
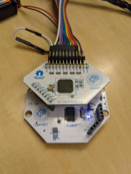
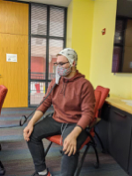
Research Thrust RT-4
Test prototype enhanced robotic systems using Collaborative Human-Machine Interfaces (CHMI) in manufacturing environments. Thrust 4 tests structurally embedded electronic systems and controlling software in real-world applications. Testbeds include co-robotic part manipulation and assembly, manufacturing of MEMS devices, and machine shop and maintenance environments. Feedback from this task will be essential to optimizing and enhancing components from Thrusts 1 – 3.
Objective 4.1.1: Determine to what extent CHMIs impact operator learning and transferring of manufacturing skills. (Popa, Nasraoui)
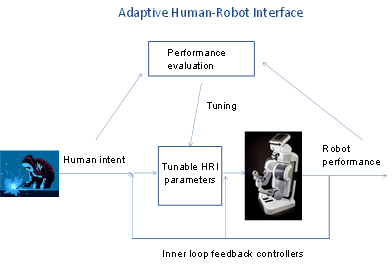
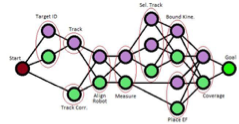
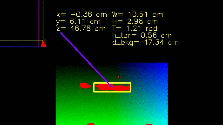
Objective 4.1.2: Optimize CHMI design, placement, networking and performance. (Popa)
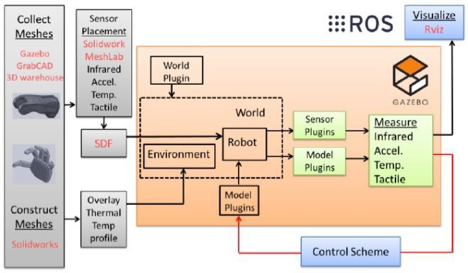
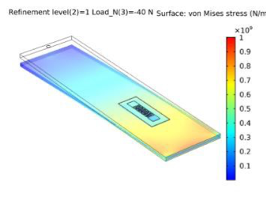
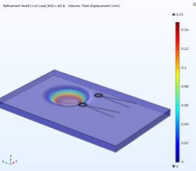
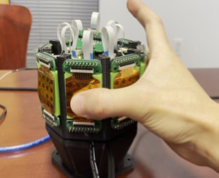
Objective 4.1.3: Measure how CHMIs improve performance compared to conventional interfaces in terms of metrics (e.g., task completion time, quality, safety, resource utilization, etc.) (Popa, Welch)
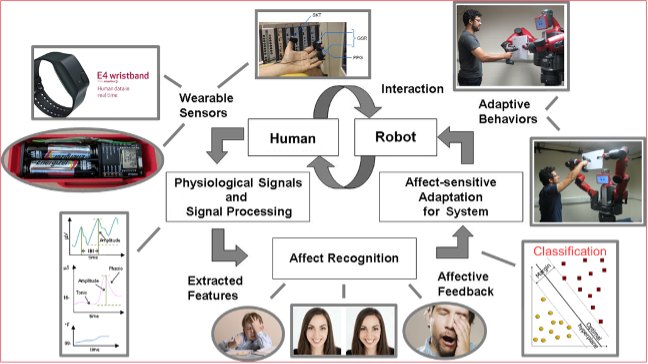
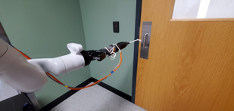
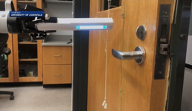
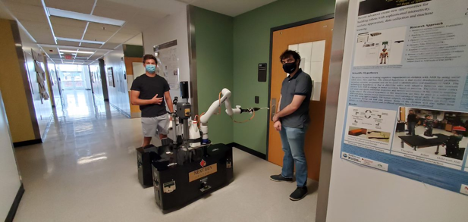
Objective 4.2.1: Manufacture SE structures using microrobotic and precision manufacturing systems and address challenges resulting from in-situ printing, bonding and packaging of hybrid micro-devices and systems containing silicon, glass, metals and polymers. (Popa, Harnett)
Objective 4.2.2: Evaluate the integration of structural electronics into microrobotic components. (Popa)
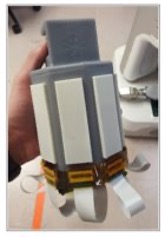
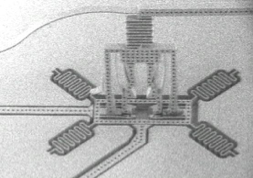
Objective 4.2.2: Evaluate the integration of structural electronics into microrobotic components. (Popa)
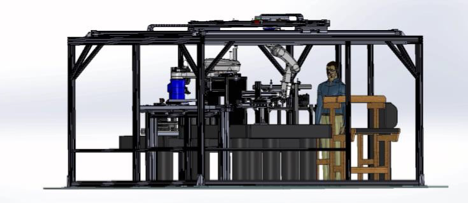
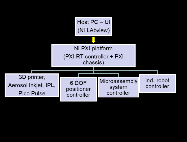
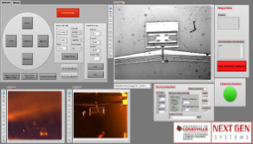
Objective 4.3.1: Develop machine-learning algorithms for failure prediction and condition-based maintenance data from SE structures. (Nasraoui, Popa)
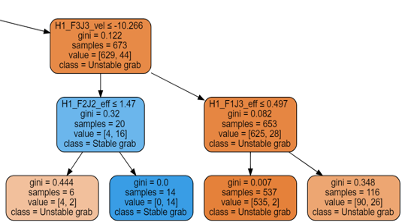
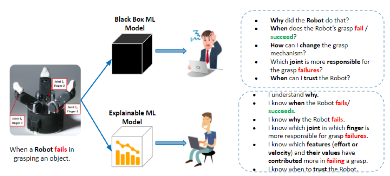
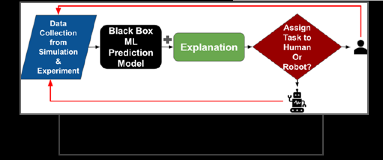